Research is heating up to achieve greater fundamental understanding of the mechanism of ferroelectricity in hafnia-based materials, a crucial step in the development of next generation devices. New findings from the University of Groningen (RUG) in the journal Science define the key role of oxygen for greater miniaturization potential and structural stability beyond that of standard ferroelectric materials used in low-power memories. Electron microscopy and MAX IV’s NanoMAX beamline have illuminated the nature of polarization in thin films of hafnium zirconium oxide for ferroelectronics.
“The actual motion of oxygen came as a surprise,” said Pavan Nukala, now Assistant Professor at the Centre for Nanoscience and Engineering at the Indian Institute of Science (IISc) in Bangalore, and lead author of the study. “With indirect experiments, people knew oxygen and oxygen vacancies were important in ferroelectricity but were ignoring that it could be directly intertwined with it in some sense. It was understood that oxygen is moving, but still the ferroelectricity [must be] happening because of other reasons. The direct connection was missing how oxygen helps in ferroelectricity.”
The researchers aimed to understand the mechanism of the ferroelectric polarization on a model capacitor designed with hafnium zirconium oxide (HZO), a silicon compatible, non-toxic material. Utilizing a high-resolution transmission electron microscope (TEM) at RUG and application of voltage, they expected to see a change in polarization, with slight oxygen displacement in the hafnia layer. What they directly observed instead was increased polarization with a charge motion—a large cycling or reversible mass migration of oxygen from the bottom electrode to the top electrode, both of which act as sinks and sources of oxygen.
Ferroelectricity is a property of spontaneous electric polarization present in certain materials which can be reversed by applying an electric field. This switching process is much akin to the flipping of north and south poles in a magnet by applying an opposite magnetic field. Today the technology is commonly used in low-power devices such as computer memories, pyroelectric sensors, and piezoelectric transducers. A known device limitation is loss of ferroelectric charge, and therefore device efficiency, with decreasing size. This makes it incapable for microelectronic applications.
The HZO capacitor is part of a new materials class of ferroelectrics, which exhibit several beneficial characteristics, including greater atomic stability with thinner dimensions—enabling the potential for nano applications, a robustness even with increased external temperature, and most pointedly, a reversible flow of oxygen ions through the middle material layer found to enhance polarization switching.
The evolution
The research project has its roots in a multi-year collaboration between MAX IV scientist Dina Carbone and Beatriz Noheda, a ferroelectricity expert, and materials science professor at the University of Groningen in the Netherlands. Their aim was to test ferroelectricity concepts using a nanobeam synchronised with external electric field—an idea based upon earlier work by Carbone. A full team at the University of Groningen formed, led by postdoc Pavan Nukala, who’s work came in focus with transmission electron microscopy at RUG, and X-ray diffraction measurement at NanoMAX beamline at MAX IV Laboratory.
Pinpointing the success of the international collaboration, the act of scientists reaching out to work with colleagues and user groups, simply by being scientist with research interest, enabled the study to be realized.
In this sense, scientists at MAX IV enrich the research environment as well as fulfill the highly technical support function that beamline users require.
Defining a mechanism
The study was not a first pass, but a deeper dive into the nature of ferroelectric materials for Nukala. As part of a 2018 Nature study, he and colleagues successfully grew thin film samples of hafnium zirconate in single crystal form with the desired ferroelectric properties, namely polarization. Central questions remained however, which drove the research further. Why were there polarization switches, and how it was robust with reducing size?
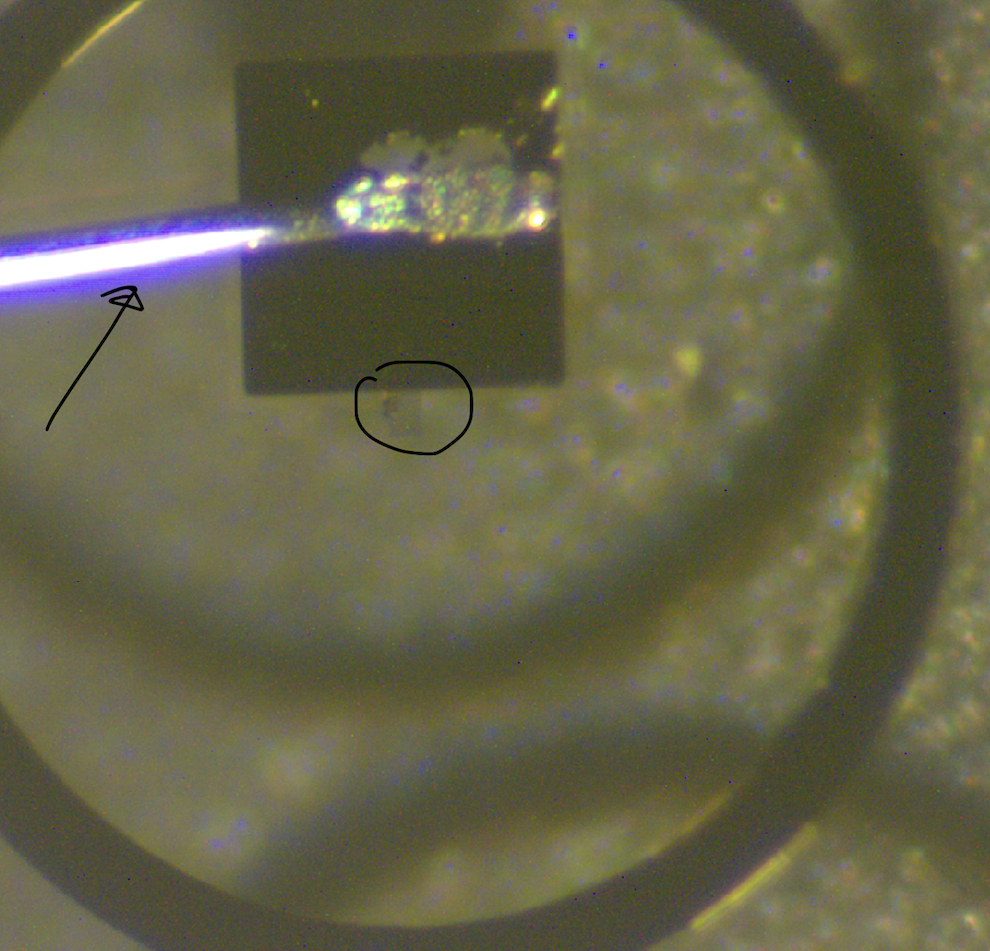
To test these questions, the group constructed a model system consisting of two electrodes and a middle layer of hafnium zirconium oxide. Nukala and Majid Ahmadi, an expert with in situ TEM technique, endeavoured to image the structure initially using the microscopy in real time with voltage changes to see how atoms move. Essentially, looking for how the oxygen lattice would be displaced by the hafnium lattice to determine polarization.
The theory was tested with both an oxygen-reactive top electrode of lanthanum strontium manganite (LSMO) and non-reactive electrode of gold (Au). Results confirmed much greater efficiency with LSMO. The opportunity to analyse the system structure with X-ray nanoprobe at MAX IV came later.
A call for more data brought the MAX IV team of Dina Carbone, scientist Danny Mannix from the European Spallation Source ERIC (ESS) and NanoMAX scientist Alexander Björling onboard the experimental side. With RUG-supplied samples, they conducted remote experiments for the research group, which was unable to travel to Lund due to pandemic restrictions. The diffraction data served as an independent, essential source of confirmation for the TEM findings of Nukala and colleagues.
“The films (samples) had very sharp interfaces, which give a clear signature in the diffraction signal. We saw these features disappearing [on samples with the Au electrode] after electrical cycling. All these fine features, and the Bragg peak, changed on the detector. I did not expected any irreversible structural variation of the films themselves or of the interfaces, or just any phase transition,” said Carbone.
This was proof that oxygen was actually moving across the HZO layer, and that the crystal structure of the material itself undergoes a phase transition. This fact clarified that the oxygen voltammetry and ferroelectric switching are intertwined in this material.
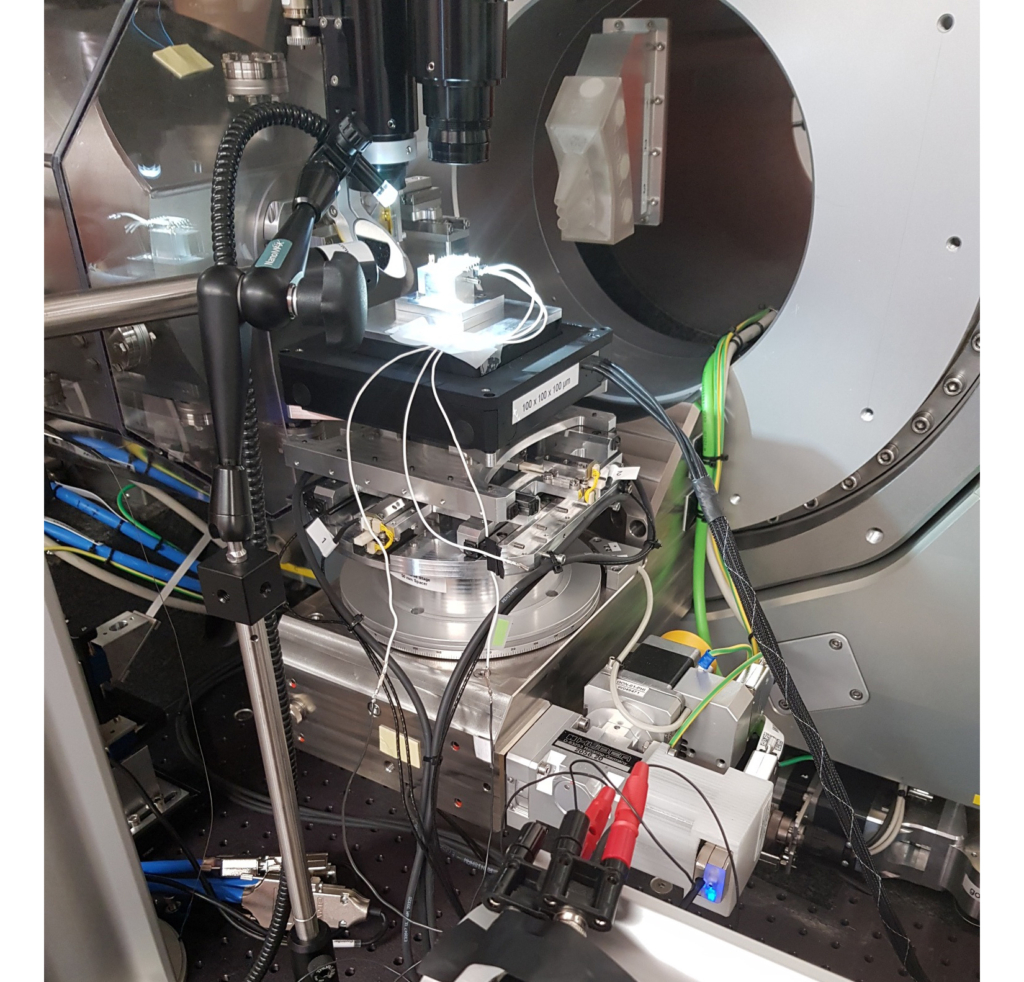
The MAX IV group measured roughly 400 crystal grains in one shot, providing highly statistically relevant data complementary to TEM. The beamline delivered fast detection and data synchronization necessary for high temporal resolution.
“X-rays bring complementarity in time and in space to TEM data. Because the grains in the sample are so small, even a small nano-beam can provide high statistical relevance. X-rays give direct information about the crystallinity of the sample, which is what changes in ferroelectrics upon the application of an electric field, and it can do it in a real time. It’s a very sharp tool to analyse these devices,” explained Carbone.
Next steps for Nukala, who now leads a research team at IISc in India, will be to further define oxygen voltammetry and the polarization switches causing ferroelectricity.
“There is also a design principle aspect to consider,” said Nukala. “Why not test different materials which exhibit properties like hafnium dioxide? Other oxygen conducting materials with oxygen reactive electrodes. You can create selection criteria for materials for new ferroelectrics in the future.”