The PRISMAS PhD programme offers challenging and exciting research projects within the following areas
healthy planet
healthy people
clean energy
sustainable technologies
accelerator science
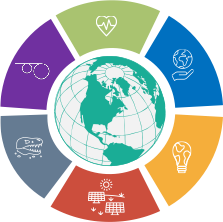
PRISMAS RESEARCH AREAS AND PROJECTS
The PRISMAS Programme had two Recruitment Calls, and the final recruitment call will take place on July-August 2024. The 1st Call was open in March-April 2023 and for the 2nd Call the application period was in September – October 2023.
Below, there is the list of four open positions during the 3rd call (open during July-August 2024).
PRISMAS POSITIONS OFFERED IN THE 3rd RECRUITMENT CALL
HEALTHY PLANET
Project title: | Chlorine Surface Activation Mechanism on Wildfire Smoke Particles and Its Relevance to Stratospheric Ozone Depletion |
PRISMAS Research Area: | Healthy Planet |
Supervisor: | Dr. Xiangrui Kong |
Partner University: | University of Gothenburg |
Wildfires have become increasingly severe and frequent, and it is discovered that wildfire smoke can activate background chlorine in the stratosphere and deplete the protective ozone layer, as seen during the 2020 Australian wildfire. In this project, the uptake and activation of chlorine species on simulated wildfire smoke particles will be
investigated by APXPS, environmental molecular beam and chamber experiments. In the APXPS experiments, the uptake, chemical fate, and depth profiles of chlorine species on smoke particles will be studied. Since this mechanism is significant in stratospheric conditions, the experimental conditions require a wide range of relative humidity and temperatures. This requires the development of a new cryo-surface holder at the SPECIES beamline, which will be carried out within the project. Complementary molecular beam chamber experiments will be conducted to validate the surface processes characterized using APXPS and to assess the importance of surface mechanisms in the atmosphere.
Project title: | Aerosol particle surface characterization in-situ for enhanced atmospheric science |
PRISMAS Research Area: | Healthy Planet |
Supervisor: | Assoc. Professor Axel Eriksson |
Hosting University: | Lund University |
Particle surfaces play an important role in the atmosphere as sites for droplet/crystal formation and growth, and by enabling heterogenous chemistry. The number of particles and their physicochemical properties governs cloud formation and properties. Processes occurring at/near aerosol particle surfaces are an integral part of the workings of our atmosphere, and investigation of these processes is essential to climate research. To this end we have recently developed an Aerosol Sample Delivery System (ASDS) for in-flight measurement of aerosol particle surfaces using synchrotron radiation and photoelectron spectroscopy. ASDS is highly complementary to real time particle bulk measurements by aerosol mass spectrometry (AMS). Together they enable comprehensive real-time measurement of aerosol constituents, providing new and essential information to further our understanding of atmospheric processes. In addition to climate perturbations, air pollution causes massive suffering and socioeconomic costs. We will target a key process underlying adverse health outcomes; lung deposition, using AMS in controlled human exposure to “background” (long-range transported) air pollution. Hence, the successful candidate will be working with both climate and health impacts from air pollution, using cutting edge measurement techniques to understand the underlying aerosol processes. Instrument development for ASDS and AMS experiments, which share many interesting technical challenges, is a central part of the coming work.
CLEAN ENERGY
Project title: | Using magnetoionics and x-ray scattering to investigate energy materials under in-operando conditions |
PRISMAS Research Area: | Clean Energy |
Supervisor: | Associate Professor Germán Salazar Alvarez |
Partner University: | Uppsala University |
Figure 1. Changes in the magnetic response (magnetisation and resonance) of the particles as a function of ion (lithium) insertion.
The magnetoionic control of materials properties is a novel approach to investigate and tune model and commercial magnetic nanomaterials used in energy applications. In this project, we aim at exploring the correlation between magnetic response through ferromagnetic resonance (FMR) or x-ray circular dichroism (XMCD) and the structural and morphological changes on nanoparticle morphology (SAXS) and crystal structure (WAXS) during lithiation under operando conditions. The simultaneous study and analysis on multiple length scales from such complex samples will provide a new in-situ tool in monitoring battery performance and potentially help to enlighten and to avoid possible failure mechanisms. We expect that we will provide with both a sample environment that can be useful to other users of MAX IV but also that we will contribute to the understanding of battery performance in nanomaterials with a comprehensive approach. Possibly, the sample environment can be used in additional beamlines.
SUSTAINABLE TECHNOLOGIES
Project title: | Identifying Active Sites in Electrochemical Ammonia and hydrocarbon Synthesis via In Situ APXPS and XAS |
PRISMAS Research Area: | Sustainable Technologies |
Supervisor: | Prof. Jiayin Yuan |
Partner University: | Stockholm University |
Constant increase in global population requires large-scale synthesis of NH3 as a feedstock to fertilizers. Reducing N2 to NH3 in aqueous media under ambient conditions and an external voltage, i.e. electrocatalytic nitrogen reduction reaction (ENRR), has been identified as a potential solution. During ENRR, NH3 is produced by activation of N2 and using water as H2 source. Transition metal nitrides have emerged as potential catalysts for ENRR following the Mars-van-Krevelen (MvK) mechanism. But recent results suggest the surface formation of metal oxynitride, which is against previous reports. The project aims to identify key mechanistic steps during ENRR on metal nitrides by monitoring the oxidation state of metal and the potential oxygen formation. This will be achieved by using in situ ambient-pressure X-ray photoelectron spectroscopy (APXPS), X-ray absorption fine structure (XAFS) spectroscopy at MAX IV. It will be generalized to electrochemical synthesis of hydrocarbons.
Below all the positions are listed, which have been open during the 1st and 2nd recruitment calls.
PRISMAS POSITIONS OFFERED IN THE 1st and 2nd RECRUITMENT CALLS
ACCELERATOR SCIENCE
Project title: | Generation of ultra-short light pulses with Accelerators |
PRISMAS Research Area: | Accelerator Science |
Supervisor: | Dr. Francesca Curbis |
Partner University: | Lund University |
Please see a detailed project description here: PhD Project overview_Curbis.pdf
The project aims to generate, develop and study future concepts in the generation of ultra-short pulses in linac based sources. A number of different techniques have been proposed involving very strong electron pulse compression, Free Electron Laser (FEL) based techniques or a combination of compression and FEL schemes. The proposed project will start by exploring the capabilities in current accelerator and FEL systems. With focus on electron beam pulse compression and how the beam properties can be retained and requirements on suitable diagnostics. From this, new concepts for even shorter pulses, 100s of attoseconds, using FEL techniques will be studied and developed.
HEALTHY PLANET
Project title: | HYDROTRANS – Migration of geologically stored hydrogen in bedrock |
PRISMAS Research Area: | Healthy Planet |
Supervisor: | Prof. Mikael Sjödahl |
Hosting University: | Luleå University of Technology |
Despite its upcoming importance as storage reservoirs in the future Hydrogen society, the migration of H2 through the Earth’s crust is poorly understood and more detailed understanding of transport and interaction mechanisms is required to meet safety requirements concerning geological storage of hydrogen in populated areas. Simulations and permeability measurements need experimental validation to perform well and the current approach is to utilize X-ray microtomography scans for detailed mapping of the microstructure and subsequently couple the structure with flow simulations. Lab-based X-ray microtomography (Zeiss Xradia 620 Versa) will be used as a step-in system and to prepare for measurements at MAX IV. By the end of this project we expect to have developed a test environment for detailed studies of gas permeability. Eventually, experiments will be performed at ESS where the combination of X-rays and neutrons promises good contrast of both H2 and the matrix.
Project title: | In situ SAXS and XANES studies to probe the structure and chemistry of nanocellulose based water treatment materials |
PRISMAS Research Area: | Healthy Planet |
Supervisor: | Prof. Aji P Mathew |
Partner University:
PRISMAS Student: |
Stockholm University
Senuri Kumarage |
Water treatment is an emerging application for nanocellulose, driven by its high surface area, versatile surface chemistry and nanostructured morphology. The current project will aim to answer different research questions related to structural and chemical aspects of nanocellulose based water treatment using Max IV facility. We will focus on i) orientation and interfacial structure during 3D printing of nanocellulose hydrogel filters ii) chemical states during in situ synthesis of nanocellulose based hybrids and iii) structural changes in nanocellulose based hybrids during water treatment. Another important outcome of this project will be the establishment of long-term collaborative networks between SU, MAX IV, RISE, Alfa Laval AB, and the training of scientists in the collaborative environment.
Project title: | The role of fungal communities in controlling mobilization of organic carbon (OC) and iron (Fe) from forest soils |
PRISMAS Research Area: | Healthy Planet |
Supervisor: | Prof. Emma Kritzberg |
Partner University:
PRISMAS student: |
Lund University
Antonios Myridakis |
This project aims to synergize forestry management with sustainability. The goal is to understand how fungal communities influence mobilization of Fe and OC from forest soils. In the background are increases in export of Fe and OC to surface waters resulting in severe OC loss. Coniferous afforestation contributes to these trends, e.g. mobilization of Fe and OC is enhanced under mature spruce forests. This may link to the fungal community, where brown-rot fungi dominate wood decomposition in coniferous forests. Many brown-rot fungi secrete extracellular metabolites that reduce FeOOH, generating Fe2+, which then produce highly reactive hydroxyl radicals that drive decay of organic matter. We expect that differences in fungi-induced biogeochemistry impact which components of wood that are degraded and remain in the soil. Synchrotron-based imaging and spectroscopy of wood and soil solution will monitor the degree of morphological and chemical change during fungal ingrowth. By integrating experimental and observational efforts we can reveal which process/es underpin transformations and fluxes observed in the field.
Project title: | Food proteins from plant sources: from structure and interactions to tunable assembly |
PRISMAS Research Area: | Healthy Planet |
Supervisor: | Associate professor Felix Roosen-Runge |
Partner University: | Lund University |
Plant seeds present promising opportunities to replace animal-derived food proteins with a more sustainable, healthy and affordable protein source. However, the difference of seed proteins to typical animal proteins requires novel approaches to create comparable food properties. The central challenge is a holistic process to extract the plant proteins in a way optimizing the potential for subsequent tunable structure formation.
We aim to apply modern scattering and microscopy techniques to
- study structure and mutual interactions of food proteins as resulting from different extraction protocols from plant seeds, and
- explore subsequent assembly processes and resulting hydrogel properties.
A comprehensive structural characterization using small-angle X-ray scattering (SAXS) presents the core of the project, using advanced modes such as SEC-SAXS to study polydisperse solutions, time-resolved SAXS to monitor assembly processes and scanning SAXS to explore spatial structural variations.
Project title: | How could oxidation state and local structure of chromium affect strategies for phosphorus recovery |
PRISMAS Research Area: | Healthy Planet |
Supervisor: | Associate professor Nils Skoglund |
Partner University: | Umeå University |
Phosphorus is identified as a critical raw material and its recycling is crucial in for a sustainable society. Recycling includes phosphorus-rich ash fractions or biochars as potential fertilizers but the chemistry of other elements must be understood.
This PhD-project is focused on how the local chemical environment of chromium, Cr, in such ashes or biochars may impact phosphorus recovery.
Cr in fertilizers is regulated both with respect to total concentration (<60 mg/kg) and oxidation state (<2 mg/kg of Cr(VI)) as its toxicity depends on its oxidation state. The complex matrix of ash fractions and biochars makes accurate determination of oxidation states for chromium near-impossible with conventional analysis.
Balder beamline at MAX IV enables analysis of Cr oxidation state in ashes and biochars relevant for phosphorus recovery by X-ray absorption spectroscopy (XAS), which combined with X-ray diffraction and fluorescence gives new insights in the chemistry of Cr in these materials.
Project title: | Closing the Loop: Chemical Speciation using XAS a Key for Safe Secondary Use of Materials |
PRISMAS Research Area: | Healthy Planet |
Supervisor: | Assoc. Professor Jenny Rissler |
Hosting University:
PRISMAS Student: |
Lund University
Evelina Gorjatsova |
To meet sustainability goals, a transition into circular economy is urgently needed. Therefore, previously overlooked residual streams need to be used as raw materials. However, the knowledge about the effects on the environment and human health of such transition remains very limited.
The toxicity, classification, and use of many inorganic residual streams are in many cases determined by the chemical form of metals occurring in low concentrations (<1%) e.g., Cu, Zn, Cr, and Sb. X-ray absorption spectroscopy (XAS) has been shown to be an excellent tool for studies of the chemical forms of trace metals in complex matrixes typical for these inorganic residual streams such as fly- and bottom ashes from waste incineration, or steel slag. It is also an excellent tool investigating the chemical forms of metals in particles emitted to air and water during the recycling processes and secondary use of these materials. And the method is even stronger if combined with XRF.
The project focuses on chemical forms of trace metals in inorganic residual streams and in the metals emitted to air (aerosols) during handling and using the materials. Also, on the fate of the toxic compounds after emission. The main method to be used is X-ray absorption spectroscopy (including both XANES and EXAFS), but also combined with 2D-mapping by XRF, all available at the Balder beamline at MAX IV.
With this project, knowledge will be generated about the nature of these materials and how they can be safely processed and used. It will also increase the understanding of emissions to air related to using the materials, and the fate of toxic compounds in biological systems.
Project title: | Spectroscopic and geometric characterization of high-valent dinuclear metalloprotein intermediates |
PRISMAS Research Area: | Healthy People, Healthy Planet |
Supervisor: | Prof. Martin Högbom |
Partner University: | Stockholm University |
If we could mimic nature’s use of metals for harvesting sunlight, energy conversion, and chemical synthesis it would eliminate the need for fossil fuels and greatly increase the possibilities of green chemistry. These reactions are based on challenging redox reactions by high-valent metal clusters in proteins. To understand and mimic this chemistry it is of central importance to know the geometric and electronic structures of the metal core as well as the protein ligand environment for these reactive intermediates.
In this project we combine serial X-ray crystallography with X-ray spectroscopy (XAS/XES) on proteins in-solution and in-crystal to determine electronic and atomic structures of key intermediates in enzymes utilizing dinuclear metal sites for some of the most challenging catalysis in nature, for example methane monooxygenase and ribonucleotide reductase. The results will be of interest for both basic and applied science in health and biomimetic catalysis.
Project title: | Uncovering the thermal and mechanical degradation mechanisms of coated ultrahard cutting tools for sustainable machining applications |
PRISMAS Research Area: | Healthy Planet |
Supervisor: | Adjunct Professor Rachid M’saoubi |
Hosting University: | Lund University |
To reduce waste, power consumption and enhance the operational safety of machined nickel alloy components used in gas turbine and wind turbine power generation applications, the development of sustainable machining technologies is relying on the use a new generation of ultrahard cubic boron nitride (PcBN) based cutting tool materials combined with high temperature and wear resistant multilayer PVD nitride coatings. This to address the need to replace traditional tooling solutions currently based on WC-Co cemented carbide where Tungsten and Cobalt are classified as critical raw materials (CRM). The optimization of such new cutting material solutions requires the development and application of relevant characterization methods to study in real time the microstructural dynamics of the PcBN/coating degradation and related mechanisms under controlled conditions of thermal and mechanical loads close to those encountered during standard operating conditions.
Accordingly, this project aims to focus on three main goals:
- Develop a high-temperature heater for in-situ X-ray study of the thermo-oxidative degradation of different PcBN/coating compositions.
- Subsequently investigate the impact of thermal degradation on the RT micromechanical properties and damage tolerance of the tool/coating interfaces via in-situ indention testing under synchrotron X-rays.
- Develop software for data analysis, in particular for scanning XRD / WAXS.
The combination of in-situ experiments at the selected Max IV beamline(s) with post-degradation characterization of the materials using HR-SEM/HR-TEM methods will give a much deeper understanding of these novel ultrahard coated tool hopefully eliminating the need of critical raw materials.
HEALTHY PEOPLE
Project title: | Structure-guided design of antiviral agents targeting coronaviruses |
PRISMAS Research Area: | Healthy People |
Supervisor: | Prof. Jens Carlsson |
Partner University:
PRISMAS Student: |
Uppsala University
Philip Ullmann |
The goal of this PRISMAS project is to develop and apply methods that can accelerate development of antiviral and anticancer drugs. We will use structure-based drug discovery to identify inhibitors of viral proteases from SARS-CoV-2 and DNA glycosylases involved in cancer. Computational design of novel chemical libraries combined with crystallographic fragment screening will identify starting-points for inhibitor development. The fragment libraries will be designed to enable hit elaboration by using commercial libraries containing billions of compounds. By using this approach, inhibitor development based on structure-based design will be faster than ever before. We will also use machine learning combined with virtual screens to search for inhibitors in the largest commercial chemical library available (>34 billion molecules). By identifying relevant regions of chemical space with machine learning, we will improve the efficiency of virtual screening and identify potent enzyme inhibitors. We anticipate to identify potent inhibitors of both viral proteases and DNA glycosylases and their effects will be evaluated in cell assays.
Project title: | Development and applications of quantum refinement for time-resolved crystallography |
PRISMAS Research Area: | Healthy People |
Supervisor: | Prof. Ulf Ryde |
Partner University:
PRISMAS Student: |
Lund University
Gayathri Yuvaraj |
X-ray crystallography has traditionally been the prime source of structural information of biological macromolecules. Such information is essential for the understanding of biomacromolecules and it is mandatory for any rational modification of these molecules, e.g. by mutagenesis or drug development. Recently, the development of powerful X-ray sources has opened the opportunity to collect series of structures in dynamic events or reactions using serial crystallography methods. A challenge for these methods is to interpret the data. Since synchronisation of the dynamic event is never perfect, the collected data typically reflect a mixture of structures, e.g. the reactant, product and intermediate states of a reaction, and it is a challenge to sort out what is really seen for the relatively poor resolution of biomacromolecules. Normally, the structures are interpreted as a mixture of states with non-unity occupancies, using empirical restraints to ensure that the structures make chemical sense. In practice, the results are often biased by what the crystallographer hope to see. Therefore, there is a need for more strict procedures for the refinement of time-resolved crystallographic structures. We will employ the quantum-refinement procedure developed in our group, which provides an ideal solution for mixtures of several states. When the electron density is ambiguous, quantum mechanical calculations give an unbiased and accurate interpretation of the density. The method will be combined with kinetic modelling of the reaction to provide an unbiased interpretation of the structure. An important part is to make the method available for any crystallography user at MAX IV.
Project title: | Visualization of vulnerable plaques by complementary use of energy dispersive X-ray phase contrast tomography and conventional histology. |
PRISMAS Research Area: | Healthy People |
Supervisor: | Assoc. Prof. Martin Bech |
Partner University: | Lund University |
Today, myocardial infarctions (heart attacks) and strokes are the largest cause of mortality in the world. Early identification of patients with vulnerable plaques is key to prevent plaque rupture. However, interpretation of clinical diagnostic images from ultrasound and MRI can be difficult. Improvements in diagnostic methods will allow for faster characterisation of vulnerable plaques and lead to better treatment. Therefore, it is significant and still unexplored to examine the potential of using multiple X-ray imaging techniques including X-ray phase imaging for the diagnosis of vulnerable plaques.
The clinical standard for characterisation of explanted plaques is histology, where the tissue sample is sliced to thin sections, stained and imaged in a microscope. Unfortunately, in this process, important information about three-dimensional morphology is lost in this process. Recent studies with synchrotron radiation have allowed us to image 3D structures such as e.g. the vasa vasorum of a carotid plaque.
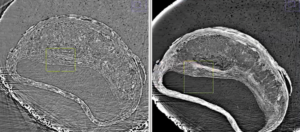
Our goal is to establish an imaging routine for carotid plaques from the bio-bank, using both a custom-built micro-CT in our own lab, and synchrotron radiation at MAX IV. We will use the DanMAX beamline to image the regions of interest in the carotid plaque, using synchrotron based micro-CT. Subsequent, the samples will be imaged with conventional histological methods, for gold standard comparison.
In this project, we will combine phase-contrast x-ray imaging with mono-chromatic and spectral micro-CT for volumetric element mapping in the carotid plaque.
Project title: | Radiosensitization for radiation therapy – X-ray induced fragmentation in DNA. |
PRISMAS Research Area: | Healthy People |
Supervisor: | Prof. Carl Caleman |
Partner University:
PRISMAS Student: |
Uppsala University
Ouassim Hocine Hafiani |
Radiation therapy is commonly used in cancer treatment, in which the main mechanism for killing cancer cells is DNA double-strand break. One problem in radiation therapy is the damage of healthy tissue. A possible solution to this is radiosensitization of tumor cells, i.e. to introduce high Z atoms into the target area. By tuning the radiation to an absorption edge of the high Z atoms, this would lead to a much more localized dose. By studying prototype biomolecules with high Z atoms using synchrotron radiation the details of these processes can be illuminated, i.e., fragmentation and the link to x-ray or Auger emission. The newly commissioned ICE setup allows pinpointing fragmentation arising from specific ionization events, and correlating these. The TRISS electrospray ionization source for biomolecules combined with the ion-trap is another essential piece of equipment for this project which both enables delivery of single biomolecules.
Project title: | Liquid-liquid phase separation mediated by phosphorylated intrinsically disordered proteins |
PRISMAS Research Area: | Healthy People |
Supervisor: | Prof. Marie Skepö |
Partner University: | Lund University |
The liquid-like properties of membranelles compartments enables them to rapidly assemble, disassemble, and concentrate components. Through liquid-liquid phase separation (LLPS) cells have evolved a process through which a transient and high level of spatiotemporal control of biochemical processes can be achieved. Intrinsically disordered proteins/regions (IDPs/IDRs) are important mediators of LLPS. The function of the former can be regulated through phosphorylation, and then induce changes in conformation, dynamics, and intra- and inter molecular interactions. Phosphorylated IDPs have received increased attention in recent years and their role in LLPS has not yet been completely understood. The aim of this project is to understand the underlying physics and role of phosphorylation on LLPS induced by IDPs. For this purpose computer simulations on both the atomistic and coasre-grained level will be used in combination with SAXS, including stop-flow technique, approximately a ratio of 75:25. This project is in collaboration with Astra Zeneca, Göteborg, Sweden, and the Co-SAXS team at MAX IV.
Project title: | Tracking ATP-Dependent Protein Dynamics |
PRISMAS Research Area: | Healthy People |
Supervisor: | Assoc. Prof. Magnus Andersson |
Partner University: | Umeå University |
Please see a detailed description of the project here: PhD Project overview_Andersson.pdf
Project summary:
Proteins rearrange their structures according to predefined patterns that are encoded into the amino acid sequence, and hence have developed throughout evolution.
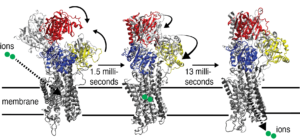
Determination of such structural dynamics and the corresponding time scales is critical to understand the biological function of proteins. In this project, we will develop a time-resolved X-ray solution scattering approach at the CoSAXS beamline to track ATP-dependent protein dynamics in real time. The structural interpretation will be obtained using supercomputer-based molecular dynamics simulations. We aim to determine kinetics and structural transition states of ATP-driven P-type ATPase membrane proteins. In particular, we will characterize regulation by membrane lipids and protein internal domains. The results will show structural rearrangements involved in regulation of ion transport, identify the time scales involved, and potentially provide better understanding of associated diseases.
Project title: | Time resolved studies of Urocanate Reductase – a Novel Microbial Enzyme Producing Imidazole Propionate |
PRISMAS Research Area: | Healthy People |
Supervisor: | Prof. Karin Lindkvist |
Partner University: | Lund University |
Urocanate reductase (UrdA) is an unexplored bacterial enzyme with to date very few published reports. UrdA was discovered in 2012 by recognising that it had the novel activity of converting urocanate to imidazole propionate (ImP). Interestingly, ImP was recently discovered to contribute to the pathogenesis of type 2 diabetes, thus the activity of UrdA is suggested to contribute to the development of type 2 diabetes and therefore a potential drug target.
The Lindkvist lab recently determined the X-ray structure of UrdA (Nature Commun. 2021). However, to fully understand the details of the urocanate reduction mechanism we aim to utilize time-resolved X-ray crystallographic studies. In addition, as UrdA has been shown to be the only enzyme to produce imidazole propionate, it is a potential therapeutic target to treat patients with type 2 diabetes and thus development of inhibitors targeting UrdA is also within the scope of the project
Project title: | Structure-based fragment screening targeting Cancer |
PRISMAS Research Area: | Healthy People |
Supervisor: | Prof. Pål Stenmark |
Partner University:
PRISMAS Student: |
Stockholm University
Julian Marius Ludäscher |
Cancer cells have an increased demand for DNA synthesis and DNA repair compared to normal cells and are therefore highly reliant on enzymes involved in these processes. This can be exploited by developing inhibitors that stop the activity of these enzymes. We will use structure-based fragment screening to develop potent inhibitors that target these enzymes, which cancer cells depend on. In recent years, structure-based fragment screening has been established as a key method to identify low affinity binders that can be developed into promising lead compounds. Billions of compounds can now be rapidly synthesized at low cost by chemical suppliers. This dramatically increases the possibilities to quickly expand and modify initial fragment hits into potential drugs. We will develop novel inhibitors and aim to bring them all the way to clinical trials. To succeed with this in an academic setting, many groups with diverse expertise have to work together.
- Gad H, et al. (2014) MTH1 inhibition eradicates cancer by preventing sanitation of the dNTP pool. Nature.
- Svensson LM, et al. (2011) Crystal structure of human MTH1 and the 8-oxo-dGMP product complex. FEBS Lett 585(16):2617-2621.
- Carter M, et al. (2015) Crystal structure, biochemical and cellular activities demonstrate separate functions of MTH1 and MTH2. Nature Commun 6:7871.
- Rudling A, et al. (2017) Fragment-Based Discovery and Optimization of Enzyme Inhibitors by Docking of Commercial Chemical Space. J Med Chem 60(19):8160-8169.
Project title: | Structure and dynamics of solid-sate formulations of biologics |
PRISMAS Research Area: | Healthy People |
Supervisor: | Prof. Vitaly Kocherbitov |
Partner University: | Malmö University |
The mechanism behind stabilization of solid-state formulations of biologics by amorphous carbohydrates and the relation to hydration is still under debate after decades of research.
The goal is to study interactions of proteins and probiotics with excipients and residual water in solid-state formulations. We propose SAXS/WAXS experiments for studying the effect of substitution of water by carbohydrate excipients in formulations of biologics. We will investigate structural changes in proteins and probiotics in the presence of excipients during combined heat and moisture treatment. Moreover, we propose to use X-ray imaging for investigation of structure and distribution of components in solid-state formulations of proteins and probiotics. The results obtained in this project will be used to optimize the design of solid-state formulations of biologics.
CLEAN ENERGY
Project title: | Operando X-ray spectroscopy of semiconductor nanostructure devices |
PRISMAS Research Area: | Clean Energy |
Supervisor: | Assoc. Prof. Rainer Timm |
Partner University:
PRISMAS Student: |
Lund University
Nishant Patel |
Please see a detailed project description here: PhD Project overview_Timm.pdf
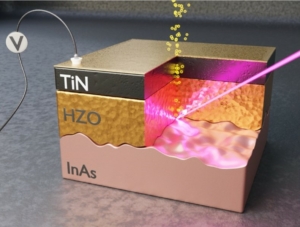
Today’s electronic devices are downscaled to a size of a few nm, and their device performance is typically determined by materials science properties of atomically thin surfaces and interfaces. This is even more valid for complex next-generation device structures such as tunnel field effect transistors (FETs), ferroelectric FETs, or resistive memories, which are strongly needed for realizing neuromorphic networks and energy-efficient internet-of-things applications.
In this project, we will study interfaces and understand physical mechanisms of such novel semiconductor devices, even by in situ experiments during device operation. We will use X-ray based spectroscopy methods at MAX IV and other European synchrotron facilities, complemented by lab-based microscopy techniques. This will be realized in close collaboration between the Division of Synchrotron Radiation Research and the Nano Electronics group at Lund University as well as researchers at MAX IV, especially the FlexPES beamline.
Project title: | Combining spectroscopy and diffraction for operando studies of complex oxides |
PRISMAS Research Area: | Clean Energy |
Supervisor: | Assoc. Prof. Lindsay Merte |
Partner University:
PRISMAS student: |
Malmö University
Philip Miguel Kofoed |
Materials incorporating multiple metal elements are active components in catalysts and electrocatalysts used in energy applications, but their complexity poses challenges for characterization under operando conditions. Hard X-ray spectroscopy provides element-specific information in gas and liquid environments, but the measurements can be difficult to interpret. Simultaneous diffraction measurements can help disentangle overlapping spectral features, and use of diffraction signals to collect spectroscopic information allows detailed analysis of chemical states at different sites in a crystal structure, though an advanced technique called DAFS. In this project, we will combine grazing-incidence XAFS, XRD, and DAFS at the Balder beamline to study the structures of 2D mixed-metal oxides under gas and electrochemical conditions. The ultimate goal is to relate the dynamic atomic-scale structure of these materials to their performance, enabling development of better catalysts in the future.
Project title: | Bridging the gap between ultrafast and steady-state: Nanosecond optical pump and X-ray probe spectroscopy for chemical, bio-inorganic and materials sciences |
PRISMAS Research Area: | Clean Energy |
Supervisor: | Prof. Philippe Wernet |
Partner University: | Uppsala University |
Controlling catalysis at the molecular level, at the level of atoms and electrons, is key to finding new ways to convert the energy of the sun into storable forms of energy. This requires a fundamental understanding of the photo-induced physical and chemical processes in molecules and materials and this is what we aim at. Within this project we will develop time-resolved X-ray spectroscopy capabilities with novel data-acquisition and detection schemes employing modern detectors and high-speed counting electronics. With our European collaborators and partners, we will work on photochemical C-H activation and photocatalytic CO2 reduction and watch in real time of chemical transformations how the energy of the sun is transformed into chemical energy.
Project title: | Resolving microsecond nanoscale fluctuations with X-ray Photon Correlation Spectroscopy at MAX IV |
PRISMAS Research Area: | Clean Energy |
Supervisor: | Asst. Prof. Foivos Perakis |
Partner University:
PRISMAS student: |
Stockholm University
Iason Andronis |
Capturing nanoscale fluctuations and ion mobility in electrolyte solutions can provide new insights in the design of clean energy storage and conversion systems. This project aims to develop and implement X-ray Photon Correlation Spectroscopy (XPCS) for probing nanoscale fluctuations of electrolyte solutions at MAX IV.
The goals are to (a) implement data acquisition from a new microsecond detector at MAX IV,(b) integrate XPCS data analysis pipelines and (c) demonstrate proof-of-concept experiments for probing nanoscale fluctuations and ion mobility in electrolyte solutions. The PhD student will learn about detector technology and be involved in X-ray experiments at MAX IV, as well as at other X-ray synchrotrons worldwide. Knowledge of data science analysis and high-performance programming methods is a key skill that the student will develop during this PhD, with broad applicability both within the academic and industry sectors.
Project title: | Soft X-ray spectroscopy study of molecular semiconductors for durable organic photovoltaics |
PRISMAS Research Area: | Clean Energy |
Supervisor: | Prof. Ellen Moons |
Partner University: | Karlstad University |
Please see a detailed project description here: PhD Project overview_Moons.pdf
Organic solar cells (OSC) have recently reached power conversion efficiencies up to 18% mainly thanks to the synthesis of new high-performance electron-donor molecules and non-fullerene electron-acceptor molecules. The remaining challenge is to increase the lifetime of OSCs. Photodegradation, in particular photo-oxidation in presence of oxygen and water vapor, of the molecular semiconductors that make up the photoactive layer is a major concern.
To study the photo-induced degradation of the components, we will, first, study intentionally exposed solution-coated thin films of selected conjugated molecules and their blends to simulated sunlight in air for increasing lengths of time. The changes in composition and electronic structure will be measured by valence band and core-level photoelectron spectroscopy (XPS) and near-edge X-ray Absorption Fine structure (NEXAFS) spectroscopy at C1s-, N1s-, O1s and other relevant X-ray absorption edges, as well as by resonant photoelectron spectroscopy. The latter is a unique technique to probe the contributions of specific parts of the molecule to the valence band structure, which is available at the FlexPES beamline at MAX IV. The 2D resonant emission maps will reveal which moieties are affected by the photodegradation, providing guidelines for the design of more photostable materials.
Secondly, we will use ambient-pressure X-ray photoelectron spectroscopy (AP-XPS) to follow selected degradation processes in-situ in controlled gas (mixture) environments. For this purpose, we aim at using an ambient pressure beamline, such as SPECIES at MAX IV. In-situ measurements will reveal the degradation kinetics and its dependence on the environment.
The outcome of this study will contribute to a better understanding of the electronic structure of these state-of-the art molecular semiconductors and the photodegradation mechanisms that limit the operational lifetime of OSCs. This understanding will provide guidelines for mitigation of photo-induced degradation and pathways towards more durable organic solar cells.
Project title: | Event-averaged and time-resolved ambient-pressure XPS as a new tool to study catalysis |
PRISMAS Research Area: | Clean Energy |
Supervisor: | Assoc. Prof. Jan Knudsen |
Partner University: | Lund University |
Please see a detailed project description here: PhD project overview_Knudsen.pdf
Project summary:
Understanding the atomic level behaviour of working catalytic surfaces is a key challenge for design of novel catalysts. Even for what appears to be steady state conditions, the catalyst structure and chemical composition – and thus reactivity and specificity – fluctuates in time in response to the instantaneous gas-mixture, pressure and temperature. Therefore, methods that can access the temporal behaviour of a catalytic system with ms-µs time-resolution during ongoing reactions must be established.
In this PhD project we will develop advanced instrumentation and methods for time-resolved in-situ measurements of catalyst materials using gas-composition, pressure, and temperature pulse pertubations. Combining this methodology with ambient pressure x-ray photoelectron spectroscopy we will focus on two key reactions: Oxidation of CyHx molecules and CO2 reduction. Catalyst structure dynamics with focus on segregation and oxidation and how this affect catalyst function will be studied.
Project title: | Time-resolved APXPS for the investigation of atomic layer deposition |
PRISMAS Research Area: | Clean Energy |
Supervisor: | Prof. Joachim Schnadt |
Partner University: | Lund University |
Atomic layer deposition (ALD) is one of the most important technologies for thin film growth, in the semiconductor device technology, but increasingly also in areas such as catalysis, fuel cells, batteries, etc. ALD is based on alternating short exposures on the ms to s timescale of a support material to different precursors that adsorb and react in a self-limiting manner. Using operando spectroscopy in the form of ambient pressure XPS (APXPS) and ambient pressure XAS (APXAS) and theoretical modelling we will investigate the surface chemistry underlying ALD. We will use the SPECIES and HIPPIE beamlines, and we will implement the method of stroboscopic operando spectroscopy recently developed at MAX IV for catalysis investigations (Knudsen et al., Nature Communications 12 (2021) 6117) for ALD. In this way, we will be able to gain unprecedented time resolution in the observation of ALD. The experimental studies will be combined with theoretical modelling of the studied ALD processes.
Project title: | Topology of Ultra Thin Metal Films on Semiconductors |
PRISMAS Research Area: | Clean Energy |
Supervisor: | Assoc. Prof. Hanmin Zhang |
Partner University: | Karlstad University |
The aim of the project is to study the fundamental physics of topological behaviors of metal films on semiconductors. In such systems, film morphology depends on topological coupling between films and substrates. The quantum confinement of the systems is characterized with the interface spin coupling between the film and the substrate.
One topic is the Ag layers grown on Sn/Ge(111)-√3*√3, which has a transition from √3*√3 to 3*3 at low temperature. The STM images showed that even at room temperature the quasi 3*3 and 2√3*√3 phases co-exist on the surface upon deposition. These data clearly point towards a topological coupling of the spin-polarized surface of Sn/Ge(111)-√3*√3with incoming Ag atoms. Other closely related systems are Pb/Ge(111)-√3*√3, Sn/Si(111)-√3*√3, and Pb/Si(111)-√3*√3.
The project is mainly of fundamental character, but may have an impact on the applications in ambient environment. The main experimental techniques are STM, spin-resolved ARPES, and NEXAFS.
Project title: | Nanoscale domain fluctuations in functional materials from X-ray photon correlations |
PRISMAS Research Area: | Clean Energy |
Supervisor: | Martin Beye |
Hosting University: | Stockholm University |
Devices in information technology rely on tailor-made materials that often have fluctuating domains of different phases enabling energy-efficient functionality.
X-ray photon correlation spectroscopy (XPCS) with soft X-rays will be applied to quantum materials, mainly at MAX IV, but also in Berlin or Hamburg, Germany, although only MAX IV will enable access to unprecedented time scales, due to novel detectors and high coherent flux. The project aims to (i) establish the method with the most advanced detectors, (ii) study domain fluctuations at the transition between 2D films to 3D bulk pieces of an antiferromagnet and (iii) drive fluctuations in analogues of high-temperature superconductors to shed new light on the underlying mechanisms and provide another piece to this long-standing puzzle. Besides skills in ultra-high vacuum experiments with synchrotron light and solid-state physics, this project also teaches capabilities in large-scale data analysis and high-performance programming.
SUSTAINABLE TECHNOLOGIES
Project title: | Elucidating time-resolved formation and assembly of lignin nanoparticles in the presence of metal ions |
PRISMAS Research Area: | Sustainable Technologies |
Supervisor: | Assoc. Prof. Mika H. Sipponen |
Partner University:
PRISMAS Student: |
Stockholm University
Prajakta Manal |
Trees grow to considerable heights owing to the presence of lignin that reinforces plant cell walls and provides the living plant with water transport and barrier properties. Lignin nanoparticles (LNPs) form from amphiphilic lignin molecules with carboxylic acid and phenolic hydroxyl groups. These molecules can chelate and reduce metal ions, which is useful in various applications like water purification and ion exchange systems. However, it is unclear how multivalent metal ions dynamically interact with lignin and how this affects LNP aggregation and network structure formation. This project aims to study the impact of metal ions on LNP formation using time-resolved SAXS measurements in levitating drops and microfluidic channels at CoSAXS. By doing so, the project seeks to establish a mechanistic understanding and control over the formation of multifunctional lignin-metal hybrid particles and their utilization in bio-based composite materials.
Project title: | In situ X-ray spectroscopy to unravel electrochemical CO2 reduction mechanism to sustainable fuels and chemicals. |
PRISMAS Research Area: | Sustainable Technologies |
Supervisor: | Dr. Sergey Koroidov |
Partner University: | Stockholm University |
Using CO2 as a feedstock to produce energy resources and chemicals instead of emissions to the atmosphere would reduce our impact on global warming and dependence on fossil-based carbon sources. Copper is the only known metal that electrochemically converts CO2 to hydrocarbons, but the process has yet to be fully understood. Our study aims to develop a fundamental understanding of the reaction mechanism, which intermediates are involved in different reaction steps, and how they bind to the catalyst. We focus on different formulations of copper as an electrode. For unknown reasons, some of these may give a high selectivity for the desired product ethylene over the unwanted greenhouse gas methane. What determines this and how it can be optimized is a significant challenge for the attack. With the methods we now have available, this is within reach. This project thus has the potential to mitigate our impact on climate change and secure our access to energy in the fossil-free future.
Project title: | In-situ corrosion in complex alloys |
PRISMAS Research Area: | Sustainable Technologies |
Supervisor: | Assoc. Prof. Rebecka Lindblad |
Partner University:
PRISMAS Student: |
Uppsala University
Varun Raj Damerla |
Corrosion causes materials to degrade and economic and environmental factors therefore motivate a search for corrosion resistant materials. A specific area of interest is metallic materials found in fuel cells and electrolysers for hydrogen production, which are exposed to a harsh environment where corrosion is a risk. The understanding of the boundaries between materials and surrounding environments at the atomic scale and under electrochemical control is essential to develop new corrosion resistant materials. In this project photoelectron spectroscopy and related techniques will be used to follow corrosion at an atomic level while it is taking place.
The project is a collaboration between Alleima, Swerim and Uppsala University and is linked to the HIPPIE beamline at MAX IV. The project will deliver a fundamental understanding of the corrosion process in coatings of complex alloys, which is particularly important for the design of new types of alloys with high corrosion resistance.
Project title: | Multiscale dynamics of nano-cellulose materials using unique Rheo-SWAXS techniques at MAX IV |
PRISMAS Research Area: | Sustainable Technologies |
Supervisor: | Prof. Karen Edler |
Partner University: | Lund University |
Cellulose is one of the most abundant materials on the planet, and cellulose fibrils are strong, lightweight and biodegradable. Key to their future use as rheology modifiers in e.g. paints or cosmetics, in cellulose packaging films or in 2D or 3D printing inks, is control of their hierarchical structure. Elucidating the multiscale behavior in flow as well as when formed into films remains a challenge. This project will combine rheology, polarized light imaging and small angle X-ray scattering (SAXS) and dynamic mechanical analysis with SAXS in new simultaneous measurements, developed recently at MAX IV. Shear-aligned nanocrystals give structural colour, so printing cellulose suspensions could avoid use of toxic inks on biobased packaging. We will also study nanocellulose films: film flexibility, strength, and response to humidity are key for packaging. This project will be the first to apply these two unique techniques to the understanding and development of novel nanocellulosic materials.
Project title: | Orientation of cellulose nanofibers in spun filaments and effect of moisture studied in-situ WAXS |
PRISMAS Research Area: | Sustainable Technologies |
Supervisor: | Prof. Kristiina Oksman |
Partner University: | Luleå University of Technology |
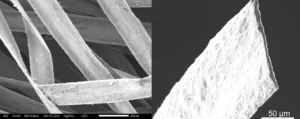
Cellulose filaments based on cellulose nanofibers are of interest for sustainable composites. These filaments have a unique micro-tape shape which is advantageous compared to traditional circular fibers because of the more efficient packing and their ability to make very thin laminates. The aim of the project is to discover if these filaments can act as structural reinforcement and understand how the nanofibers are oriented along the filament axis and how the orientation varies across the filament width to be able to model their mechanical properties. Furthermore, understand how moisture affects the filament structure and mechanical properties. The aim is to use WAXS with a highly focused beam to study the orientation of the cellulose nanofibers across the filament and to use in-situ mechanical tests in moisture as well as to use WAXS and X-ray tomography to evaluate the effect of moisture on the structure and properties.
Project title: | Using NanoMAX to understand Additive manufacturing of rare-earth-free permanent magnets |
PRISMAS Research Area: | Sustainable Technologies |
Supervisor: | Prof. Martin Sahlberg |
Partner University:
PRISMAS Student: |
Uppsala University
Daniel Rainer |
The principal aim of the project is to exploit possibilities generated by additive manufacturing (AM) to design, characterise, predict and control anisotropic properties in metallic materials, with emphasis on rare-earth-free permanent magnets. Preferred directional grain growth and high cooling rates are two important parameters in creating anisotropic properties in technologically important material classes.
In particular, we will investigate the use of laser powder bed fusion AM to control texture development during manufacturing. We plan to combine a highly controlled AM-process with advanced crystallographic methods, using X-ray (and neutron) probes to establish best practise in the manufacture and processing of materials with desired anisotropic properties.
Here, the proposed project will employ hard x-ray nanoprobe imaging, diffraction and x-ray magnetic circular dichroism (XMCD) to measure phase transitions and anisotropy in AM produced RE-free permanent magnets.
Project title: | Misfit strain detection in precipitate hardening alloys by Bragg coherent diffraction imaging |
PRISMAS Research Area: | Sustainable Technologies |
Supervisor: | Assoc. Prof. Martin Fisk |
Partner University: | Malmö University |
The next generation polycrystalline nickel-base superalloys for application in highly demanding environments, such as aero-engines, or heat exchangers will not only reduce the fuel consumption but also the amount of NOx emission. One major problem is, however, that high-temperature resistance superalloys frequently end-up in a composition that are sensitive for crack formation. It may occur during heat treatments, or in the manufacturing process where intragranular γ’-precipitates formed. Initially these precipitates are fully coherent, or semi-coherent with the matrix of the alloy, which may result in misfit strains. And consequently, if the density of the nucleated precipitates is larger than the matrix phase, all individual grains are contracted, and severe strains in the grain boundaries appear. This phenomenon is known as Strain Age Cracking (SAC) for the industry.
Here, the proposed project aims to increase the understanding of SAC by in-situ measuring the strain field of individual γ’-precipitates embedded in a metallic γ-matrix by Bragg coherent diffraction imaging for various temperatures, precipitate sizes, and alloy compositions.
The experimental results are important, not only for understanding the role of misfit strains on precipitation but also for calibrating and validating numerical models acting on the same length scale, e.g., phase field crystals. These results, from the nanometer scale, will be used in a larger campaign where the aim is to understand the SAC phenomenon on several length scales, from the individual particles on the nanoscale up to the micrometer scale where the cracking takes place.
The main supervisor will be Associate Professor (docent) Martin Fisk, Materials science and applied mathematics, Malmö University.The project will jointly be with NanoMax.
Project title: | Quantum properties of direct-Chemical vapor Deposited two-dimensional (2D) heterostructures |
PRISMAS Research Area: | Sustainable Technologies |
Supervisor: | Associate Professor Venkata Kamalakar Mutta |
Hosting University: | Uppsala University |
Two-dimensional (2D) material van der Waals (vdW) heterostructures exhibit extraordinary quantum traits for next-generation nanoelectronics, spintronics, and optoelectronic applications. The project aims to investigate the quantum (electronic, phononic, and magnonic) properties and phenomena in novel directlychemical vapor deposited 2D material heterostructures and their functionalized defect-passivated systems, and develop innovative devices.
Here we explore a new direct growth of 2D material heterostructures, for example, semiconducting MoS2 on graphene. Recently, we have innovated the growth of composite 2D heterostructures by nucleationdefined chemical vapor deposition. In this project, we specifically aim to probe the electronic structure of these new quantum materials via advanced x-rays spectroscopic tools to achieve the following objectives:
- Elucidate the band structure and surface charge transfer in directly CVD-grown 2D heterostructures of MoS2 over graphene and 2D magnets (Chromium Telluride) over graphene.
- Unveil the role of spin-orbit coupling and its influence on the electronic and magnonic (involving magnetic 2D materials) band structure in 2D heterostructures.
- Develop a mechanistic picture of the defect passivation by chemical treatments on CVD-grown 2D materials.
These objectives will provide first-time results that will impact the understanding of the properties of the directly grown 2D heterostructures and act as a guide to engineering their properties for efficient next-generation optoelectronic and spintronics devices, which will be innovated with these quantum materials.